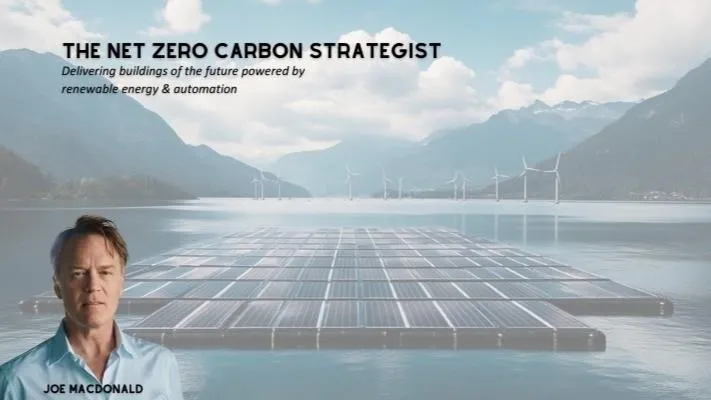
Achieving Net Zero Today: An In-Depth Exploration of Solar, Wind, and Battery Storage Across All Available Land and Sea
Introduction
The accelerating threat of climate change demands immediate and transformative action. The Intergovernmental Panel on Climate Change (IPCC) has underscored the urgency of limiting global warming to 1.5°C above pre-industrial levels, necessitating a rapid shift to net-zero carbon emissions by around 2050. This ambitious target requires a fundamental overhaul of our global energy systems, moving away from fossil fuels toward renewable energy sources. This exploration delves into a hypothetical yet critical scenario: achieving net-zero emissions today by relying exclusively on solar and wind energy, complemented by battery backup and storage, utilizing every available land and sea resource.
This comprehensive analysis examines the scale of infrastructure required, technological advancements necessary, environmental and social implications, and the practical feasibility of such an undertaking. By incorporating case studies and current projects, we aim to illuminate the challenges and opportunities inherent in this monumental energy transition.
Global Energy Demand and the Scale of Renewable Energy Deployment
Understanding the enormity of transitioning entirely to solar and wind energy with battery storage begins with grasping the current global energy landscape. As of 2021, the world's total primary energy consumption stood at approximately 583 exajoules (EJ), equivalent to around 162,000 terawatt-hours (TWh). This energy powers every facet of modern life, including electricity generation, transportation, heating, industry, and more. Fossil fuels—coal, oil, and natural gas—dominate this consumption, accounting for about 84% of the total. Renewable energy sources, while growing, still represent a relatively small fraction.
To achieve net-zero emissions, it's not enough to replace fossil fuel-based electricity generation. A significant portion of energy consumption in sectors like transportation and heating relies directly on fossil fuels. Electrifying these sectors would substantially increase global electricity demand, potentially doubling it to approximately 300,000 TWh annually by 2050. Meeting this demand solely with solar and wind power requires deploying renewable energy infrastructure on an unprecedented scale, necessitating vast investments and global coordination.
Solar Energy Potential
Solar power presents an immense opportunity due to the sheer volume of solar radiation the Earth receives. The sun delivers enough energy to the planet in one hour to meet global energy needs for an entire year. Harnessing even a fraction of this energy could revolutionize the global energy supply.
Modern photovoltaic (PV) panels have made significant strides in efficiency, with commercial panels achieving efficiencies between 15% and over 22%. Accounting for average solar irradiance and efficiency losses due to factors like weather and nighttime, the average power output of solar panels is about 10 watts per square meter. To generate 150,000 TWh annually from solar energy—assuming solar provides half of the required renewable energy—we would need approximately 4.1 million square kilometers of solar panels. This area is slightly larger than India and represents about 2.7% of the Earth's land surface.
Technological innovations are enhancing the efficiency and reducing the costs of solar power. Perovskite solar cells, for instance, offer the promise of higher efficiencies and lower production costs. Recent laboratory developments have achieved efficiencies exceeding 25%, and companies like Oxford PV are working toward commercializing perovskite-silicon tandem cells for mass production. Bifacial solar panels, which capture sunlight from both sides, can increase energy yield by up to 30%, particularly effective when installed over reflective surfaces such as sand or snow.
Incorporating solar cells into building materials, known as Building-Integrated Photovoltaics (BIPV), allows for energy generation without additional land use. The Tesla Solar Roof exemplifies BIPV in residential applications, transforming rooftops into energy-producing surfaces. By integrating solar technology into windows, facades, and other structural elements, BIPV can significantly expand the potential for solar energy harvesting in urban environments.
Utilizing non-arable land maximizes solar energy deployment without competing with agriculture or conservation. Deserts and semi-arid regions, which receive high solar irradiance and have low population densities, are ideal for large-scale solar installations. Projects like the Noor Complex in Morocco, one of the world's largest concentrated solar power (CSP) plants, demonstrate the potential of desert solar farms. The complex harnesses solar thermal energy, storing heat for electricity generation even when the sun isn't shining, thus addressing intermittency issues.
Floating solar farms represent another innovative approach. By installing solar panels on bodies of water, such as reservoirs, lakes, and even coastal areas, land space is conserved, and the cooling effect of water can improve panel efficiency. The Huainan Floating Solar Farm in China, built on a former coal-mining region, is the world's largest floating solar project with a capacity of 150 MW. This project not only generates clean energy but also rehabilitates previously unusable land.
Case Study: The Benban Solar Park in Egypt
The Benban Solar Park exemplifies large-scale solar deployment's potential and challenges. Located in Egypt's Western Desert, Benban is one of the world's largest solar installations. With a planned total capacity of 1.8 GW, the park comprises 41 individual projects developed by various companies. The project has attracted over $2 billion in investment, much of it from international financiers like the International Finance Corporation (IFC). During construction, it created thousands of jobs, stimulating the local economy and providing employment opportunities in a region with limited prospects.
Benban contributes significantly to Egypt's goal of generating 20% of its energy from renewable sources by 2022. By reducing reliance on fossil fuels, the project helps decrease greenhouse gas emissions and promotes energy security. However, the project's scale has posed challenges, including coordinating among multiple developers and integrating the generated power into the national grid. The success of Benban underscores the importance of supportive government policies, international cooperation, and robust infrastructure in large-scale renewable energy projects.
Wind Energy Potential
Wind energy harnesses the kinetic energy of air currents, offering a mature and rapidly growing renewable energy source. Onshore wind farms have been established for decades, and advancements in turbine technology have increased efficiency and output. Offshore wind, though more recent, taps into stronger and more consistent winds at sea, offering significant potential.
Wind farms require considerable space due to the size of turbines and the need to minimize aerodynamic interference between them. Onshore wind farms typically require about 0.3 square kilometers per MW of capacity. However, the land between turbines can often be used for other purposes, such as agriculture or left as natural habitat, minimizing the impact on land use. Offshore wind farms, while not competing for land space, require substantial maritime areas. The Hornsea One project in the UK, the world's largest offshore wind farm, has a capacity of 1.2 GW and spans approximately 407 square kilometers.
Technological advancements are expanding wind energy's potential. Floating offshore wind farms allow turbines to be placed in deeper waters beyond the continental shelf, where wind speeds are higher and more consistent. The Hywind Scotland project, the world's first floating wind farm, demonstrates this technology with five turbines totaling 30 MW. Larger turbines are also enhancing efficiency; GE's Haliade-X 14 MW turbine, standing 260 meters tall with blades 107 meters long, is capable of powering 16,000 European homes annually.
Vertical Axis Wind Turbines (VAWTs), though less common, offer advantages in certain conditions. They can operate well in turbulent winds and may be more suitable for urban environments or integration into buildings. VAWTs also have potential applications on floating platforms due to their lower center of gravity.
Case Study: The Gansu Wind Farm in China
The Gansu Wind Farm, also known as the Jiuquan Wind Power Base, illustrates the possibilities and challenges of large-scale wind power deployment. As of 2020, the installed capacity exceeded 10 GW, with plans to expand to 20 GW, making it one of the world's largest wind power installations. Located in the Gobi Desert, the project takes advantage of strong and consistent wind resources.
However, the Gansu Wind Farm has faced significant challenges. The rapid expansion outpaced the development of transmission infrastructure, leading to curtailment issues where generated power cannot be delivered to where it's needed. At times, up to 40% of the wind farm's capacity was unused due to grid limitations. This situation highlights the importance of synchronizing renewable energy development with grid expansion and the integration of storage solutions to manage variability and transmission bottlenecks.
Battery Backup and Energy Storage
Energy storage is critical in a renewable energy-dominated grid to balance supply and demand and ensure reliability. Solar and wind power are intermittent, producing energy only when the sun shines or the wind blows. Storage solutions allow excess energy generated during peak production periods to be saved and used when production is low or demand is high.
Addressing daily fluctuations requires short-term storage solutions like lithium-ion batteries. These batteries have seen significant improvements in performance and cost reduction. Since 2010, the cost of lithium-ion batteries has declined by 89%, driven by advancements in technology and economies of scale. The Tesla Gigafactory in Nevada exemplifies efforts to increase production capacity, aiming to produce 35 GWh of battery capacity annually, primarily for electric vehicles but also for grid storage applications.
Flow batteries represent an emerging technology offering scalability and long cycle life, suitable for grid-scale applications. Unlike lithium-ion batteries, which store energy in solid electrodes, flow batteries store energy in liquid electrolytes contained in external tanks, allowing for easy scaling by increasing tank size. The Dalian Flow Battery Energy Storage Peak-shaving Power Station in China, with a capacity of 200 MW/800 MWh, showcases the potential of flow batteries in large-scale energy storage.
Solid-state batteries are another promising development, offering higher energy density and improved safety over conventional lithium-ion batteries. Companies like QuantumScape are making progress toward commercial viability, potentially revolutionizing energy storage with batteries that charge faster, last longer, and are less prone to fire risks.
Beyond batteries, alternative storage solutions play a crucial role. Hydrogen storage involves using excess renewable energy to produce green hydrogen via electrolysis. This hydrogen can then be stored and converted back into electricity using fuel cells or used directly as a fuel for transportation and industry. The Hydrogen Energy Supply Chain (HESC) project in Australia aims to produce and export hydrogen to Japan, demonstrating international cooperation in developing hydrogen as an energy carrier.
Pumped hydro storage remains the largest form of energy storage globally. Facilities like the Bath County Pumped Storage Station in the United States, with a capacity of 3 GW, store energy by pumping water to a higher elevation during periods of excess generation and releasing it to generate electricity during peak demand. Compressed Air Energy Storage (CAES) facilities, such as the Huntorf plant in Germany, store energy by compressing air in underground caverns, which is later expanded through turbines to generate electricity.
Case Study: Hornsdale Power Reserve in Australia
The Hornsdale Power Reserve, known as the Tesla Big Battery, demonstrates the transformative impact of battery storage on grid stability and efficiency. Located in South Australia, the facility was initially built with a capacity of 100 MW/129 MWh and later expanded to 150 MW/193.5 MWh. The battery provides essential grid services, such as frequency control and rapid response to outages, significantly enhancing grid stability.
In its first two years of operation, the Hornsdale Power Reserve saved consumers over $150 million by reducing reliance on expensive gas peaker plants and mitigating the impact of network disruptions. The project's success has spurred further investments in battery storage in Australia and worldwide, illustrating the economic and operational benefits of integrating large-scale battery storage into the grid.
Utilizing All Available Land and Sea
Maximizing renewable energy deployment requires strategic use of land and maritime areas, balancing energy production with environmental protection and social considerations. Large-scale projects must navigate complex land use issues, ecological impacts, and community acceptance.
Environmental constraints are a significant consideration. Renewable energy projects must avoid critical habitats and minimize disruptions to biodiversity. Environmental impact assessments are essential to identify and mitigate potential negative effects. For instance, wind farms in the North Sea are carefully designed to avoid interfering with bird migration routes and marine life. Moreover, offshore installations can sometimes benefit marine ecosystems by acting as artificial reefs, enhancing local biodiversity.
Land use conflicts can arise when energy projects compete with agriculture, conservation, or residential areas. Integrating renewable energy generation with other land uses offers solutions. Agrivoltaics, the practice of combining solar panels with agriculture, allows for dual land use. Jack's Solar Garden in Colorado is a prime example, where a 1.2 MW solar array coexists with crop production, providing shade that can benefit certain plants and reduce water evaporation.
Community acceptance is crucial for the success of renewable energy projects. Involving local populations in planning and offering economic benefits can foster support. The Middelgrunden Offshore Wind Farm near Copenhagen is partially community-owned, with local residents investing in and benefiting from the project. This model of community ownership increases public engagement and acceptance.
International cooperation enhances resource utilization and addresses the geographic mismatch between renewable energy resources and demand centers. Developing high-capacity transmission networks, or supergrids, allows for the efficient transfer of electricity over long distances. The proposed Euro-Asia Interconnector aims to link the power grids of Israel, Cyprus, and Greece, facilitating the flow of electricity between continents. China's Global Energy Interconnection Development and Cooperation Organization (GEIDCO) advocates for interconnected grids to optimize renewable energy use worldwide, promoting energy security and reducing reliance on fossil fuels.
Technological and Infrastructural Challenges
Implementing a global transition to renewables faces significant technological and infrastructural hurdles. Scaling up production of renewable energy technologies requires vast resources and manufacturing capacity. Critical minerals such as lithium, cobalt, rare earth elements, and copper are essential components of solar panels, wind turbines, and batteries. The World Bank estimates that production of these minerals could increase by nearly 500% by 2050 to meet demand.
Ensuring sustainable and ethical sourcing of these materials is imperative. Environmental degradation and social conflicts associated with mining activities can undermine the benefits of renewable energy. Initiatives like the Responsible Minerals Initiative work toward improving supply chain transparency and promoting responsible mining practices.
Upgrading grid infrastructure is essential to accommodate the variability and distributed nature of renewable energy sources. Traditional grids designed for centralized, controllable power plants are ill-suited for decentralized and intermittent renewable generation. Modernizing grids involves incorporating digital technologies to manage energy flows efficiently, known as smart grids. Countries like South Korea are investing heavily in smart grid infrastructure, enhancing reliability and integrating renewable energy sources.
Integrating energy storage solutions at various grid levels enhances flexibility and reliability. The California Independent System Operator (CAISO) has incorporated significant battery storage capacity to support its renewable portfolio, enabling better management of supply and demand fluctuations.
Supportive policy and regulatory frameworks are crucial for enabling the energy transition. Government incentives, subsidies, and mandates can stimulate investment in renewable energy. For instance, the U.S. Investment Tax Credit (ITC) has been instrumental in promoting solar deployment. Adjusting electricity market structures to value flexibility and storage services encourages the integration of energy storage technologies. The UK's National Grid introduced the Optional Downward Flexibility Management service to handle excess renewable generation during periods of low demand.
Economic Considerations
The financial aspects of transitioning to a renewable energy-dominated system are substantial but offer significant long-term benefits. The International Renewable Energy Agency (IRENA) estimates that achieving the energy transition requires cumulative investments of around $131 trillion by 2050. This investment spans infrastructure development, technology deployment, and system integration.
Despite the high upfront costs, renewable energy sources have become increasingly cost-competitive. The global weighted average Levelized Cost of Electricity (LCOE) for utility-scale solar PV fell by 85% between 2010 and 2020. Wind power also saw significant cost reductions, with onshore wind costs declining by 56% and offshore wind by 48% over the same period. These trends are expected to continue as technologies mature and economies of scale are realized.
Transitioning to renewable energy can stimulate economic growth and job creation. IRENA reports that renewable energy employed 12 million people globally in 2020, with potential to reach 42 million by 2050. The renewable energy sector offers employment opportunities across manufacturing, installation, maintenance, and support services.
Additionally, reducing air pollution through renewable energy adoption leads to health benefits and associated cost savings. The European Environment Agency estimates that air pollution costs the EU between €330-940 billion annually due to health impacts. Improving air quality can reduce healthcare expenditures and increase productivity by lowering illness-related absenteeism.
Case Study: Denmark's Renewable Energy Economy
Denmark provides a compelling example of how investment in renewable energy can drive economic growth and innovation. The country generates over 50% of its electricity from wind power, a result of decades of consistent policy support and investment. Danish companies like Vestas and Ørsted have become global leaders in wind technology, exporting expertise and equipment worldwide.
Denmark's commitment to renewable energy has enhanced energy security by reducing reliance on imported fossil fuels. The country's experience demonstrates how a clear policy framework, public support, and investment in innovation can lead to a successful energy transition with economic benefits.
Environmental and Social Implications
The transition to renewable energy presents both positive impacts and challenges. Environmental benefits include significant reductions in greenhouse gas emissions, essential for meeting climate targets. Renewable energy sources produce little to no emissions during operation, contributing to climate change mitigation.
Improved air quality is another major benefit. Fossil fuel combustion releases pollutants like sulfur dioxide, nitrogen oxides, and particulate matter, which have adverse health effects. Transitioning to renewables reduces these emissions, leading to better public health outcomes.
However, renewable energy projects can also have environmental impacts. Infrastructure development may disrupt habitats and ecosystems. For instance, wind turbines can pose risks to birds and bats if not properly sited. Mitigation strategies include careful site selection, technology solutions like radar detection systems, and operational adjustments.
Resource extraction for renewable energy technologies can cause environmental degradation and social conflict. Mining activities may lead to deforestation, water pollution, and displacement of communities. Addressing these issues requires enforcing environmental regulations, promoting corporate social responsibility, and supporting affected communities.
Social considerations are critical to ensuring a just and equitable energy transition. Community engagement in project planning helps address local concerns and ensures that benefits are shared. Supporting workers transitioning from fossil fuel industries through retraining and job placement programs is essential. The European Union's Just Transition Mechanism allocates €17.5 billion to support regions and communities most affected by the transition, aiming to mitigate social and economic impacts.
Case Study: The Navajo Nation's Transition
The Navajo Nation in the United States exemplifies the complexities of transitioning from fossil fuels to renewable energy. Historically, the Nation relied heavily on coal mining and power generation for employment and revenue. The closure of coal plants and mines has had significant economic impacts on the community.
In response, the Navajo Nation is investing in renewable energy projects to create new opportunities. The Kayenta Solar Facility, with a capacity of 55 MW, provides clean energy and jobs. However, challenges remain in scaling up renewable energy development, securing investment, and ensuring that projects meet the community's needs. The Navajo experience highlights the importance of tailored policies, financial support, and capacity-building to facilitate a just transition.
Feasibility and Alternatives
While achieving net-zero emissions today using only solar, wind, and battery storage is theoretically conceivable, practical challenges make it currently unattainable. The technological, infrastructural, and logistical hurdles are significant. Manufacturing capacities for solar panels, wind turbines, and batteries need substantial expansion, requiring time and resources.
Existing grids are not designed to handle 100% renewable energy and require extensive upgrades. Energy storage solutions, while advancing, are not yet capable of addressing all the intermittency challenges at the required scale. Moreover, the global coordination needed to utilize all available land and sea resources effectively is unprecedented.
Diversifying the energy mix enhances feasibility. Incorporating other low-carbon energy sources, such as nuclear energy, can provide reliable baseload power. Countries like France, where nuclear power accounts for about 70% of electricity generation, demonstrate the role nuclear energy can play in reducing emissions.
Hydropower offers significant capacity where geographically feasible. The Three Gorges Dam in China, the world's largest hydropower station at 22.5 GW, contributes substantially to China's renewable energy portfolio. Geothermal and biomass energy can also contribute, particularly in regions with suitable resources.
Reducing energy consumption through efficiency measures eases the transition. Implementing energy-efficient technologies in buildings, industry, and transportation can significantly lower demand. Demand response programs that adjust consumption patterns to match renewable generation profiles improve system flexibility.
Global coordination and supportive policy frameworks are essential. The Paris Agreement represents an international commitment to limit global warming, with countries submitting Nationally Determined Contributions outlining their plans. The United Nations Sustainable Development Goals, particularly Goal 7, focus on ensuring access to affordable, reliable, sustainable, and modern energy for all.
What Using Just WIND Would Look Like
Relying exclusively on wind energy to meet global energy demands would be a monumental challenge, necessitating the development of wind farms on nearly every viable piece of land and across all seas. The sheer scale of such an endeavor is immense, and it highlights both the potential and the constraints of this renewable resource.
Scale and Coverage
Global electricity demand is projected to reach 300,000 TWh annually by 2050. Assuming wind energy as the sole provider:
With an average capacity factor of 35% (typical for wind turbines), this would require an installed capacity of approximately 98 TW.
Onshore wind farms need around 0.3 square kilometers per MW, translating to 29.4 million square kilometers—an area nearly the size of Africa.
Offshore wind, with its higher capacity factors (~45%), would reduce land requirements but would need vast expanses of maritime space. Covering the world's seas with turbines would require careful planning to avoid critical shipping lanes, fisheries, and ecological hotspots.
Environmental and Economic Considerations
Ecological Impact: Massive onshore deployments could disrupt ecosystems, while offshore turbines, though less invasive, could affect marine life. Careful siting and innovations like quieter turbines and artificial reef designs would be necessary.
Infrastructure Challenges: Extensive grid expansion and energy storage solutions are required to manage variability and ensure consistent supply.
Economic Viability: Building turbines across all feasible areas would require trillions in investment, with significant ongoing costs for maintenance and upgrades.
Global Feasibility
The integration of floating wind farms allows for deeper offshore installations, leveraging stronger and more consistent winds. However, achieving the required scale would involve global cooperation, massive manufacturing capacity, and breakthroughs in turbine efficiency and storage technology.
What Using Just SOLAR Would Look Like
Using only solar energy to power the world would require not just terrestrial land but also floating solar panels on vast bodies of water. While the sun offers an inexhaustible energy source, the deployment scale is unprecedented.
Land and Sea Requirements
To meet 300,000 TWh of annual energy demand:
Assuming an average output of 10 watts per square meter, around 30 million square kilometers of solar panels would be needed—equivalent to the combined area of the United States, China, and Australia.
Land use conflicts could arise, necessitating innovative solutions like agrivoltaics or Building-Integrated Photovoltaics (BIPV) to minimize competition with agriculture and urban areas.
Floating Solar Panels: These could be deployed on reservoirs, lakes, and oceans, potentially covering around 10 million square kilometers of water. Large-scale offshore projects would need to account for corrosion, storm resilience, and the impact on marine ecosystems.
Technological and Environmental Challenges
Efficiency Innovations: Advanced technologies like perovskite solar cells and bifacial panels would need to dominate to reduce spatial demands and increase energy output.
Cooling and Maintenance: Floating solar panels benefit from water’s cooling effect, improving efficiency, but maintaining these installations in harsh marine environments would be costly and complex.
Ecological Impact: Offshore solar farms could affect aquatic ecosystems, including changes in water temperature and shading that might disrupt local habitats.
Economic and Logistical Considerations
The estimated cost for deploying and maintaining such expansive infrastructure runs into tens of trillions of dollars. Manufacturing, transporting, and installing panels at this scale would require global coordination and massive supply chain expansion.
Balancing Wind and Solar
Using either wind or solar energy alone to meet global energy needs would require an extraordinary transformation of the Earth's land and seas. Both approaches underscore the necessity of complementary technologies, such as battery storage, and a diversified energy mix that includes other renewable sources. While wind and solar are central to our sustainable energy future, relying solely on one would strain resources, ecosystems, and economies to their limits.
Conclusion
Achieving net-zero emissions today using only solar, wind, and battery storage across all available land and sea represents an enormous and complex challenge. It requires not only technological advancements and massive infrastructure investments but also careful consideration of environmental impacts and social implications.
Case studies and current projects demonstrate the potential of renewable energy deployment and the benefits it can bring. They also highlight the necessity of a diversified approach, integrating multiple energy sources and efficiency measures. While immediate attainment of net-zero emissions using solely solar, wind, and battery storage is impractical, these technologies are central to long-term strategies for a sustainable energy future.
By continuing to invest in renewable energy, enhancing international cooperation, and fostering innovation, we can move closer to achieving net-zero emissions. Addressing the challenges requires collective effort, but the opportunities for environmental protection, economic growth, and social well-being make the endeavor imperative. The pursuit of a sustainable energy future is not just a technical challenge but a societal one, demanding commitment from governments, industries, and individuals alike.