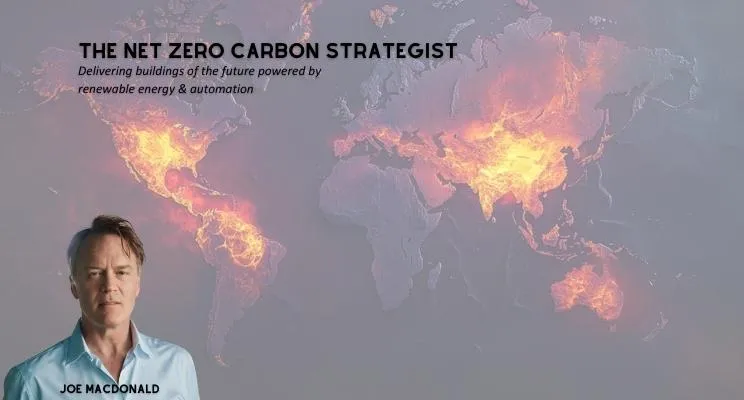
Geothermal Energy Rising: The Next Big Player in Clean Power
Across continents and energy discussions, solar and wind often dominate headlines as the flag-bearers of a clean energy revolution. Yet, beneath the Earth’s crust lies a powerful and largely untapped resource: geothermal energy. Recent findings from the International Energy Agency’s (IEA) report, The Future of Geothermal Energy, project that after solar, the clean energy source most capable of fueling tomorrow’s power grids may not be wind or hydropower—but rather the heat simmering beneath our feet.
Although geothermal plants currently make up only a tiny sliver of global electricity generation, momentum is growing rapidly. Technological breakthroughs in drilling, reservoir engineering, and advanced geothermal systems have opened doors for geothermal in countries that once assumed they lacked resources. According to the IEA, next-generation geothermal could supply up to 15% of the increase in global electricity demand by 2050, reflecting around 800 gigawatts (GW) of new capacity—enough to match the combined electricity demand of the United States and India today. Just as solar cells and wind turbines once soared from niche status to mainstream dominance, geothermal appears primed for a similar leap forward.
This article explores why geothermal is generating renewed enthusiasm, the technical breakthroughs that support its rise, the big market players, the policy gaps that still exist, and the future of an industry that aims to dig deeper into the Earth than ever before. Along the way, we will see how next-generation geothermal systems promise to provide round-the-clock, low-carbon electricity that bolsters grid stability, offers unique baseload potential, and fosters a synergy with traditional oil and gas drilling knowledge. We will also unravel the environmental, economic, and policy challenges that need to be overcome for geothermal to claim its place as the second-largest clean energy source in the decades to come.
The IEA Report and the Geothermal Upswing
When the International Energy Agency releases a report forecasting major shifts in the global energy mix, policy circles take notice. The Future of Geothermal Energy underscores the geothermal sector’s vast theoretical potential, some of which has been understood by scientists for decades. However, the new thrust of the report is that with next-generation systems (including enhanced geothermal systems, advanced closed-loop designs, and supercritical drilling methods), the potential is not only theoretical but eminently achievable—given concerted research, development, and investment.
The IEA’s data projects that geothermal could provide as much as 15% of the growth in world electricity demand by 2050, requiring up to 800 GW of additional geothermal capacity worldwide. That figure translates into an annual output comparable to the existing combined electricity demand of the U.S. and India. To appreciate the importance of that projection, note that global electricity demand is accelerating, as economic growth lifts millions of people out of energy poverty and technologies like electric vehicles and data centers multiply power needs. If geothermal’s slice of the pie grows to even a fraction of the IEA’s target, it would represent a remarkable shift in the global energy mix.
Why Geothermal: The Appeal of Constant Baseline Heat
Much of the renewed interest in geothermal flows from its unique advantage: reliability. Unlike solar, which depends on sunlight, and wind, which depends on weather patterns, geothermal power harnesses heat from the Earth’s core. Temperatures hundreds or thousands of meters below ground remain relatively constant. This consistency lets geothermal facilities run at high capacity factors. In 2023, average global utilization rates for existing geothermal plants topped 75%—whereas wind and solar often range between 15% and 45%, depending on location, weather, and other factors. As such, geothermal fits the mold of “always-on” or “firm” power, more akin to traditional fossil fuel plants or nuclear reactors in terms of reliability.
Grid operators have long sought flexible, dependable energy sources to stabilize electricity systems. In an era of heightened fluctuations from intermittent renewables, geothermal’s baseload characteristic becomes especially valuable. According to the IEA, advanced geothermal plants can also ramp up and down relatively quickly, aiding the integration of solar PV and wind by offering a stable “floor” of generation, plus the ability to adjust output when the sun is obscured or the wind fades. In other words, geothermal is not only an excellent source of continuous power but can also play a role in balancing the overall grid—crucial for the deep decarbonization of energy systems.
From Larderello to the Geysers: Traditional Hydrothermal Resources
Historically, geothermal power has been associated with places like Italy’s Larderello region, the Geysers field in California, and Iceland’s high-temperature fields. These “traditional” geothermal systems exploit naturally occurring geothermal reservoirs—where high temperatures, steam, or hot water exist close enough to the surface to be tapped with relatively simple drilling. Yet, such prime spots are scarce, located primarily in volcanic and tectonically active regions like the Ring of Fire in the Pacific. Even though these conventional resources fueled early interest and remain vital to the industry, they represent only a fraction of the Earth’s subterranean heat.
Still, big conventional developments like The Geysers in Northern California, with around 900 MW capacity, illustrate how geothermal can anchor a regional grid for decades. The Cerro Prieto field in Mexico, with nearly 1,000 MW, and plants in Indonesia, the Philippines, New Zealand, and Kenya have also shown how reliable geothermal can provide up to a quarter or more of a country’s electricity. Despite these successes, the global installed geothermal capacity remains modest, hovering around 16 GW according to some estimates—far behind hydropower, wind, or solar. That discrepancy may change if advanced systems unlock less conventional resources worldwide.
Next-Generation Geothermal: Tapping Heat Almost Anywhere
The big story in the IEA’s report and in geothermal industry circles is the array of next-generation technologies now moving from research laboratories into the field. Their main objective is to extract high-temperature heat from deep rock formations—even where there is no naturally abundant fluid or favorable geology.
Enhanced Geothermal Systems (EGS). EGS technology involves drilling deep into hot rock formations and artificially creating or expanding fracture networks, thereby increasing the permeability enough to circulate water or a working fluid. By injecting cold water into one well, letting it travel through newly enhanced fractures, and then pumping out the superheated fluid from a production well, operators can harvest heat from regions previously deemed unsuitable. Although EGS has faced challenges with induced seismicity and high drilling costs, pilot projects in the U.S., Europe, and elsewhere have demonstrated its promise.
Closed-Loop (Advanced Geothermal Systems). Another approach is the closed-loop system, where a working fluid circulates in a sealed pipe that travels deep underground, absorbing heat from the surrounding rock without relying on in-situ fluids. Such systems can potentially be installed in places with limited natural permeability or water resources. The reduced risk of fluid loss and the avoidance of hydraulic stimulation appeals to developers, but drilling and material costs remain barriers.
Supercritical Geothermal. For extremely high temperatures, sometimes encountered at depths below 5 or even 8 kilometers, water can become “supercritical,” meaning it takes on properties of both a liquid and a gas. If harnessed properly, supercritical resources can yield dramatically higher energy per well than conventional reservoirs. Research in Iceland, for instance, has sought to drill deep enough to reach supercritical zones and unlock their enormous power density.
According to the IEA, the full technical potential of next-generation geothermal—particularly when drilling goes below 8 kilometers—could theoretically meet global electricity demand 140 times over. Obviously, not all of that resource can be practically accessed or developed cost-effectively. Yet, the fundamental point is that Earth’s heat is vast, and new technologies are making it feasible for many more regions, not just the volcanic hot spots, to participate.
Market Outlook: China, the U.S., and India Leading the Charge
As with all major infrastructure, policy and investment decisions drive growth trajectories. China, the United States, and India are primed to be the largest adopters of next-generation geothermal, accounting for around 75% of new capacity additions by some estimates. Each of these nations has multiple reasons to embrace geothermal:
• China seeks to reduce air pollution, diversify away from coal, and meet ambitious clean energy targets. Large swaths of the country could tap deeper geothermal resources if cost-effective drilling emerges.
• The United States already hosts the world’s largest installed geothermal capacity (around 3.9 GW), mainly in California and Nevada. With advanced drilling technologies and abundant sedimentary basins, the U.S. could expand far beyond its traditional geothermal strongholds, especially if new policy incentives align with a push for low-carbon baseload power.
• India grapples with massive increases in energy demand amid a push to decarbonize and reduce reliance on coal. While India has historically focused on solar and wind, emerging pilot geothermal projects could prove that the country’s deep basins harbor usable heat.
Even regions not typically known for geothermal activity may see expansion. New drilling techniques have opened the possibility of harnessing resources at 3 kilometers or deeper, where temperature gradients are high enough to provide power generation or industrial heat. The IEA underscores that nearly all countries possess some level of accessible geothermal resource, if advanced drilling technologies and supportive policies converge.
Reliability, Flexibility, and Baseload Attributes
A defining advantage of geothermal over wind and solar is its ability to deliver electricity around the clock. While new energy storage systems and improved grid management have made intermittent renewables far more viable, they still can’t provide continuous output on their own. Baseload capacity—traditionally supplied by coal, gas, or nuclear—remains essential to maintaining grid stability, especially through peak demand or adverse weather.
Geothermal plants operate with capacity factors often exceeding 75%, meaning they typically run at or near full capacity throughout most of the year. Some facilities, especially in well-managed fields with stable reservoir temperatures, can exceed 90%. The global average capacity factor for geothermal is among the highest of any renewable energy source, comparable to well-managed hydropower (though hydropower can face seasonal fluctuations).
In addition to steady production, modern geothermal facilities can ramp up or down relatively quickly compared to many traditional baseload plants, providing a flexible resource for grid operators. This flexibility is crucial for mitigating the variable outputs of wind and solar—essentially allowing geothermal to act as a partner technology that anchors a low-carbon grid. Some experts argue that a high penetration of intermittent renewables will only be feasible if enough flexible baseload or storage capacity stands ready. Geothermal could fill much of that niche in multiple markets worldwide.
Environmental and Social Dimensions
Though geothermal is broadly considered a clean energy source, it does carry certain environmental impacts that must be carefully managed. Traditional hydrothermal plants can emit traces of hydrogen sulfide (H₂S), a gas known for its “rotten egg” odor and potential health hazards at high concentrations. Modern abatement technologies, however, can remove up to 99.9% of H₂S emissions, and binary cycle plants (closed-loop systems) often emit virtually zero. Another concern is induced seismicity, especially for enhanced geothermal systems that involve pressurized fluid injections. While most induced earthquakes are small, community acceptance can be hampered by fear of geological disturbances—an issue that has led to project delays or cancellations in places like Switzerland and South Korea. Regulators increasingly require seismic risk assessments and protocols, much like those deployed in the oil and gas sector for hydraulic fracturing.
Water usage and potential contamination present additional challenges. Some geothermal fluids contain minerals or trace contaminants such as arsenic, boron, or heavy metals. Proper well casing, reinjection, and closed-loop designs reduce the risk of contaminating surface or groundwater. Meanwhile, certain approaches require a significant volume of water, though typically less than many other power-generation technologies, including coal or nuclear. Careful reservoir management and reinjection ensure that long-term geothermal operations can be sustainable, preserving reservoir pressure and limiting depletion.
On the social side, local opposition can arise from misunderstandings about drilling or from concerns about land use and noise pollution. Education, transparent community engagement, and economic benefits such as local job creation go a long way in winning public support. Over time, many communities come to value the consistent royalty payments, tax revenues, and jobs that geothermal development can provide—particularly in rural areas with fewer economic options.
Cost Trajectories and Investment Opportunities
If geothermal is so promising, why hasn’t it taken off more dramatically? High upfront costs, chiefly associated with drilling and exploration, remain the single largest barrier to expansion. Drilling for geothermal can be as expensive as drilling for oil and gas, with the added risk that the target resource—sufficient temperatures and fluid flows—may not exist as anticipated. In places with uncertain geology, upfront exploration costs can dissuade private investors, given that unsuccessful wells can sink millions of dollars with no return.
However, the IEA projects that costs for geothermal power could fall by 80% by 2035, reaching around $50 per megawatt-hour (MWh). Such a decline would place geothermal on par with onshore wind and solar in many markets—particularly if it is credited for its valuable baseload and flexible generation attributes. Driving this cost reduction are:
1. Technological Innovations: New drilling methods (such as millimeter-wave drilling, plasma drilling, or advanced spallation) could reduce well costs and speed up drilling times. Enhanced Geothermal Systems, if deployed widely, would likewise cut the cost per MWh by sharing site infrastructure and increasing well productivity.
2. Economies of Scale: As more projects progress, supply chains for specialized equipment improve, labor and expertise expand, and overall efficiencies in exploration and drilling ramp up.
3. Policy Incentives: Government-backed exploration risk insurance, grants for early-phase drilling, or feed-in tariffs for geothermal electricity can lower the financial risk. In markets where geothermal is recognized as a carbon-free baseload, it may garner higher valuations or capacity payments.
The IEA suggests total investment in geothermal could reach $1 trillion by 2035, and swell to $2.5 trillion by 2050 if the technology meets its ambitious deployment targets. Such sums require mobilizing private capital, multilateral development banks, government agencies, and philanthropic sources. Importantly, the returns could be substantial if geothermal becomes a mainstream resource—offering stable, zero-emission power at a time when many countries aim for net-zero carbon grids.
Policy Gaps: The Need for Supportive Frameworks
Despite growing enthusiasm, geothermal lags behind solar and wind in terms of supportive policy environments. As the IEA points out, over 100 countries currently have policies or targets specifically for solar PV and onshore wind, while only around 30 have policies tailored to geothermal. Geothermal incentives often fail to account for the unique risk profile that can arise when drilling a $10 million well for a resource that might not pan out.
Furthermore, extended permitting and regulatory hurdles can hamper geothermal deployment. In some jurisdictions, the same site might require multiple permits from local, regional, and federal agencies, each requiring lengthy environmental reviews and public hearings. Streamlining the permitting process—while ensuring environmental safeguards—could make geothermal more attractive to private developers. Iceland, for instance, leverages a straightforward licensing process and a tradition of geothermal use, which has helped it meet a large share of its electricity and heating demand through volcanic resources.
Policymakers may also consider fiscal measures like tax credits, feed-in tariffs, or guaranteed power purchase agreements (PPAs) for geothermal projects. These instruments have proven highly effective in boosting solar and wind. If geothermal is to catch up, similar policy tools must be adapted, especially in the early stages. Over the long term, an established geothermal sector can likely compete without subsidies, provided drilling risks are mitigated and technology costs continue to decline.
The Oil and Gas Industry’s Role
A fascinating part of geothermal’s future is the role oil and gas companies could play in its expansion. Many of the technologies, workforce skills, and supply chain elements in drilling for hydrocarbons translate directly to drilling for geothermal resources. The knowledge required to operate high-temperature drilling rigs, interpret seismic data, handle well completions, and manage fluid flows is extremely relevant. As some oil and gas firms seek ways to diversify into low-carbon ventures, geothermal presents a natural adjacency.
Several pilot projects highlight this synergy. The re-use of depleted oil wells for geothermal power generation has shown promise in certain regions, though differences in temperature gradients and fluid composition may limit universal applicability. More broadly, a skilled rig workforce, specialized drilling equipment, and a wealth of subsurface data can shorten project timelines and improve success rates.
By pivoting segments of the oil and gas industry toward geothermal, the sector can leverage existing capital, infrastructure, and technical expertise. According to the IEA, this transition could generate new employment pathways, expanding the geothermal workforce up to a sixfold increase and reaching roughly one million jobs by 2030. It also provides oil and gas professionals a route to apply their experience while contributing to climate solutions—a pivot that may accelerate if carbon pricing, emission regulations, or investor pressure continue rising.
Overcoming Challenges: Technical, Economic, and Social
As with any emerging technology, next-generation geothermal systems face challenges that must be overcome for large-scale deployment:
1. Technical Complexity: Drilling at depths of 5-8 kilometers or more, possibly into supercritical zones, exposes materials to extreme temperature and pressure conditions. Tools can degrade quickly, requiring new alloys or real-time monitoring systems. The success of Enhanced Geothermal Systems depends on effectively creating and sustaining fracture networks without triggering seismic events unacceptable to local communities.
2. Exploration Risk: Geothermal exploration can feel like a gamble if the resource is not thoroughly understood prior to drilling. Advanced geophysical surveys, improved seismic imaging, and big data analytics can reduce uncertainty, but expensive “dry holes” remain a risk.
3. Financial Barriers: High capital costs, combined with uncertain resource discovery, make financing difficult. Early-phase capital is notoriously scarce, as banks and private investors often shy away from geological risk. Government guarantees, insurance mechanisms, or grants can mitigate these hurdles.
4. Regulatory Frameworks: Complex or unclear regulations cause project delays and cost overruns. Streamlining permitting while preserving environmental and safety standards is a delicate balancing act. Policy frameworks that properly value geothermal’s baseload and grid-stabilizing benefits will also be critical.
5. Social Acceptance: Induced seismicity, local environmental concerns, and a general unfamiliarity with geothermal projects can spur pushback. Robust community outreach, safety protocols, and transparent monitoring are vital. Developers who engage with local stakeholders from the outset tend to have more durable social licenses to operate.
6. Environmental Safeguards: While geothermal’s environmental footprint is relatively small compared to fossil fuels, issues like water contamination, toxic byproducts in geothermal fluids, or sensitive ecosystems around drilling sites must be managed with care. Abatement technologies for hydrogen sulfide, closed-loop systems, and water reinjection often resolve or mitigate these concerns.
In addressing these challenges, the industry is borrowing heavily from decades of progress in oil and gas drilling, hydropower licensing strategies, and the systematic rollout of solar and wind power. Innovation is fast-paced in geosciences, materials engineering, and data analytics, suggesting that obstacles can be tackled more efficiently now than in previous decades.
Geothermal in a Decarbonized Future
The global shift to clean energy is accelerating. As solar arrays proliferate across rooftops and utility-scale wind farms bloom off coastlines, planners and utility operators increasingly ask: where does baseload or dispatchable power come from? Battery storage, green hydrogen, and nuclear remain part of the mix, but geothermal possesses a major advantage—most of the needed technology already exists. It is a renewable, low-carbon resource with a proven track record stretching back over a century. Its consistency is unmatched among renewables that depend on intermittent resources.
If geothermal scales as the IEA envisions, it will profoundly reshape power systems. More communities in places like East Africa, Southeast Asia, or Central America—which lie on tectonic or volcanic regions—could leapfrog over fossil fuels entirely for their baseload needs, providing a stable backbone for sustainable development. Meanwhile, advanced EGS and closed-loop systems could unlock resources in areas previously considered unviable, including parts of Europe, North America, and Asia far from classic geothermal hotspots. The synergies with heat applications—like district heating, industrial processes, desalination, or greenhouse agriculture—may further amplify geothermal’s appeal.
For countries seeking energy independence, geothermal offers a domestic, on-site resource that can dramatically reduce reliance on imported fuels. It also balances the variability of solar and wind, potentially allowing higher penetrations of those resources. As electricity usage soars—driven by electrified transportation, heating, and industry—every region will need multiple low-carbon sources to meet the demand. By offering both baseload and flexible generation, geothermal might help close the gaps in a renewable-heavy power system.
Lessons from Existing Giants: Iceland, the Philippines, and Beyond
In looking toward the future, we can glean insights from countries that already rely heavily on geothermal. Iceland generates nearly 30% of its electricity and a high proportion of its heat from volcanic resources. The country’s success rests on supportive government policies, a culture deeply familiar with geothermal’s benefits, and robust scientific institutions that measure and map the subsurface. Despite a small population, Iceland’s experience shows the importance of social acceptance, supportive regulation, and clear economic signals.
Similarly, the Philippines and Indonesia have long tapped their geologically active regions. Today, the Philippines ranks among the top producers of geothermal electricity, powering portions of the archipelago, while Indonesia is closing in on the U.S. as the top producer. Both countries demonstrate how harnessing geothermal can reduce dependence on coal or oil imports, though each faces challenges expanding to new sites and addressing environmental constraints. Still, they serve as real-world testbeds for scaling up geothermal in emerging markets.
Kenya’s remarkable progress—expanding geothermal capacity in the Olkaria field—shows how developing nations can utilize underground heat for both electricity and direct use, lifting local economies and stabilizing grids prone to interruptions. Such examples illustrate that while technology is critical, alignment between policymakers, communities, and investors is equally vital.
A $2.5 Trillion Prospect
The road to mainstreaming geothermal may be long and fraught with hurdles, yet the payoff is potentially enormous. With estimates suggesting $2.5 trillion in global geothermal investment by 2050, the sector stands on the cusp of a generational expansion. If realized, this investment would not only generate gigawatts of clean electricity but also create a flourishing ecosystem of high-skilled jobs, from drilling engineers to reservoir modelers, geophysicists, materials scientists, and plant operators. The synergy with oil and gas skill sets could enable a relatively smooth transfer of talent as more conventional hydrocarbon basins mature or decline.
Moreover, the integration of geothermal with other renewables multiplies value. Excess geothermal heat can be used in combined heat and power (CHP) setups, direct heating for industrial processes, or community district heating systems. In regions with intense solar production, geothermal could provide night-time or cloudy-day coverage, augmenting reliability. Studies also show that geothermal’s constant output can reduce the overall cost of decarbonizing the grid, because fewer large-scale storage or peaking plants are needed.
Yet, success depends heavily on addressing the core challenges of cost, policy, public acceptance, and technical risk. Policymakers who recognize geothermal’s potential must craft frameworks that stimulate drilling and early exploration, de-risking the initial capital outlay that frequently hobbles prospective projects. International lenders and development banks can play a catalytic role by providing concessional financing or partial risk guarantees. Entrepreneurs in advanced geothermal drilling will need continued support to scale their pioneering methods, bridging laboratory breakthroughs to full commercial viability.
Conclusion: Geothermal’s Moment in the Sun (and Under the Earth)
In the race to secure clean, reliable energy for a growing world, geothermal is poised to play a starring role right behind solar power—if the sector can capitalize on current tailwinds. The resource is immense: deep beneath almost every landmass lies a heat reservoir capable of producing emissions-free electricity and heat for decades. Regions with strong policy support, skilled labor (often from the oil and gas industry), and robust geological data are already pressing forward. The reliability of baseload geothermal capacity makes it a gem for grid operators hoping to integrate large shares of intermittent wind and solar.
The IEA’s vision of geothermal capturing up to 15% of new global electricity demand by mid-century may strike some as ambitious, particularly given the sector’s small installed base today. But the rapid transformations seen in solar PV—once considered too costly for wide adoption—demonstrate how quickly technology, policy, and industry consensus can shift. Once dismissed as a niche source viable only in volcanic hotbeds like Iceland, geothermal is increasingly recognized as a global resource waiting for the right combination of scientific and economic conditions to flourish.
A $2.5 trillion investment by 2050, new drilling methods that slash costs by 80%, and a sixfold increase in geothermal-related jobs are not outlandish projections for a planet in need of firm, clean electricity. As the energy transition accelerates, geothermal stands ready to fill a major gap: delivering stable, low-carbon baseload and heat in a world leaning ever more on renewables. With concerted effort in technology, finance, and policy support, the subterranean heat that has silently powered local grids for decades may soon roar into the global mainstream.
Main Challenges in Deploying Next-Generation Geothermal Technologies
Despite geothermal’s promise, bridging the gap between scientific potential and actual large-scale deployment hinges on overcoming several significant hurdles. Unlike solar modules or wind turbines that can be tested and replicated relatively quickly, next-generation geothermal developments require long, capital-intensive projects with high geological risk. Below, we examine the main challenge areas—technical, economic, regulatory, and social—hindering the global scaling of advanced geothermal systems.
Technical Complexity and Deep Drilling Risks:
At the forefront is the difficulty of drilling to extreme depths (commonly 5 kilometers or more) to access higher-temperature zones. This challenge is magnified when targeting supercritical resources at depths of 8 kilometers or beyond. Current drilling techniques face wear and tear from hard basement rock and intense heat, driving up costs and project timelines. Innovations in advanced drilling—like millimeter-wave or plasma-based methods—could solve some of these issues, but they need further testing. Similarly, Enhanced Geothermal Systems must reliably create and sustain fracture networks in low-permeability rock, balancing reservoir performance with concerns about induced seismicity.
High Upfront Capital Costs and Perceived Risks:
Geothermal projects, particularly those employing next-generation techniques, often carry high capital expenditure. Securing financing can be challenging because investors balk at the geological uncertainty of “wildcat” geothermal wells. Weighted average costs of capital can be high, compounding the financing burden. Early-stage resource exploration, akin to oil and gas exploration, often lacks the insurance or risk-sharing mechanisms found in more mature sectors.
Regulatory Barriers and Policy Gaps:
In many countries, the regulatory environment for geothermal is inconsistent or nonexistent. Developers can face duplicative permitting at local, regional, and national levels, leading to extended project timelines. Although more than 100 countries have policies supporting solar and wind, only around 30 have strong geothermal regulations or incentives. Environmental reviews may be further complicated if the project is in seismically sensitive regions or near protected areas. Clear, transparent frameworks that streamline permitting while maintaining safety and environmental standards are essential.
Induced Seismicity and Environmental Concerns:
Pressurized fluid injection in EGS can trigger small earthquakes. While most are too minor to be felt at the surface, public opposition can arise, fueled by incidents in Switzerland and South Korea. Additionally, geothermal fluids can carry traces of heavy metals, hydrogen sulfide, or other contaminants. Proper reinjection, advanced fluid handling, and abatement systems largely mitigate these risks, but regulatory agencies and local communities demand rigorous monitoring and safety plans.
Social Acceptance and Community Engagement:
Communities unfamiliar with geothermal or skeptical due to experiences with mining or fracking may resist drilling projects. Public trust hinges on consistent communication, transparent data sharing, and visible community benefits. Developers often learn the hard way that engagement must start early in project planning, addressing concerns about noise, dust, truck traffic, and potential seismic events.
Skills and Workforce Development:
Advanced geothermal systems require specialized skills at the intersection of geoscience, engineering, data analytics, and project management. Training a new cohort of drilling engineers and reservoir modelers demands coordination between industry, universities, and governments. The shortage of expertise can slow project development, though it also presents an opportunity for regions with established oil and gas sectors to pivot into geothermal.
Market Signals and Grid Integration:
Even as technology costs drop, developers need reliable markets for the electricity and heat generated. In liberalized electricity markets, projects must compete on cost with cheaper natural gas or subsidized renewables like solar and wind. Where grid capacity is limited or policy frameworks do not reward baseload and flexibility, geothermal can be undervalued relative to variable renewables.
Despite these hurdles, the upside remains compelling. As cost trends continue downward and knowledge transfer from oil and gas intensifies, the path to widespread adoption of next-generation geothermal is becoming clearer. Governments seeking carbon neutrality by mid-century will need stable, low-carbon baseload that complements solar and wind. If policymakers create risk-sharing mechanisms, streamline regulations, and highlight geothermal’s unique grid and environmental benefits, the sector could witness a dramatic expansion. In that scenario, geothermal would no longer be the overlooked cousin of solar and wind, but rather a robust, globally distributed resource helping power economies around the clock.
Ultimately, the challenges are surmountable with strategic public-private collaboration. Addressing drilling technology, financing models, regulatory clarity, and public acceptance head-on will determine how fully geothermal energy can bloom into the second-largest source of clean power after solar. Given the Earth’s almost inexhaustible supply of heat, and the evolving technological toolkit to access it, the future of geothermal could be as bright as the star at the center of the solar conversation—except geothermal’s star burns deep beneath our feet, day and night, ready to propel the next wave of the clean energy revolution.